Motivation
Animals and robots must transition between different modes of locomotion (e.g., walk, run, climb) when moving on complex 3-D terrain with obstacles as large as themselves. While animals can transition robustly by making effective physical interaction with the terrain, robots that use obstacle avoidance (Figure 1a-c) often fail. This is because most of our mechanistic understanding is on how to generate and stabilize near-steady-state locomotion in a single mode. We do not yet understand how animals use physical interaction to make robust locomotor transitions, and how robots should do so (Figure 1d-e) in complex 3-D terrain with large obstacles.
Here, we describe our recent progress towards filling this knowledge gap by discovering terradynamic principles of multi-legged locomotor transitions. We first focused on understanding passive mechanical interactions and studied cockroaches during their rapid, bandwidth-limited escape or self-righting response and feed-forward-controlled robotic physical models. This provided a foundation for understanding sensory feedback control, and the intelligence aspect of locomotor-terrain interactions.
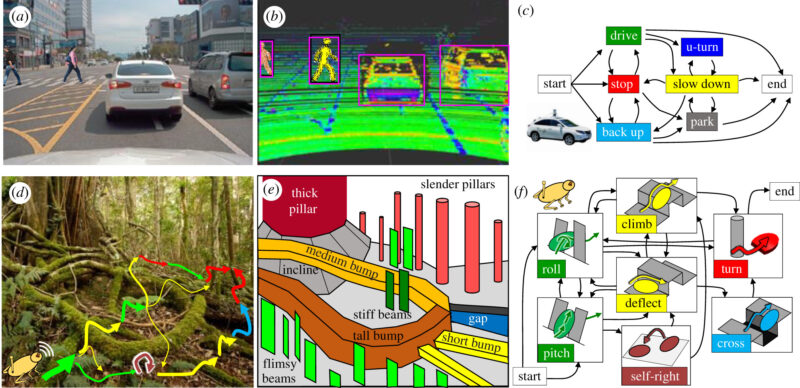
Figure 1. Multi-pathway transitions to avoid and traverse obstacles. (a) View from a self-driving car. (b) Geometric map scanned. (c) Multi-pathway driving transitions to avoid obstacles. (d) Envisioned capability of robot traversing complex three-dimensional terrain with many obstacles as large as itself. (e) Abstracted challenges from diverse large obstacles. (f) Envisioned multi-pathway locomotor transitions. Image credits: (a,b), Modified with permission under Creative Commons CC-BY license. (d) Modified with permission from Luke Casey Photography.
Methods
We abstracted complex terrain as a composition of diverse large obstacles (Figure 1d, e) that present distinct locomotor challenges (Figure 1f). We created simplified robotic physical modes of each model system to generate relevant locomotor behavior. To enable systematic experiments we created a terrain testbeds that allow controlled, systematic variation of model system properties. We developed tools to address the technical challenges in measuring locomotor transitions in complex 3-D terrain.
To understand physical principles of locomotor transitions, we created potential energy landscapes for our model systems (Figure 2a,b). We hypothesized that locomotor transitions are barrier-crossing transitions between basins of potential energy landscapes. In addition, to begin to understand transition dynamics, we also developed dynamical templates and multi-body dynamics simulations.
Principles and insights from simple model systems.
For each model system, we discovered physical principles of locomotor transitions and how transitions depend on system properties. These principles are generalizable over the relevant parameter space and led to new insights.
For all model systems, locomotor modes are stereotypical, with qualitatively similar body postural changes (Figure 2a). Transitions between modes occur stochastically and the probability of using or transitioning to a mode strongly depends on locomotor and terrain parameters.
Locomotor transitions are destabilizing, barrier-crossing transitions on a potential energy landscape (Figure 2b). For all systems, the system state in each mode is strongly attracted to a local minimum basin of the energy landscape. Because the landscape basins are strong attractors, transition from one mode to another requires escaping from a basin and falling into another by overcoming an energy barrier.
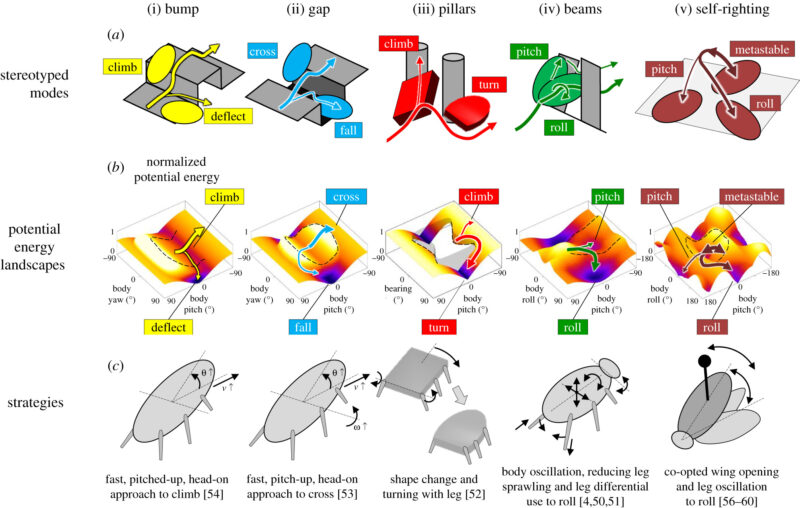
Figure 2. Modulation of locomotor transitions on potential energy landscapes via a suite of strategies. (a) Stereotyped locomotor modes of model systems. (b) Potential energy landscapes. The system is attracted to a distinct basin in each mode. A potential energy barrier must be crossed to make locomotor transitions. Black dashed curves show potential energy barriers. Arrows in (a,b) show representative system state trajectories; thicker arrows show more desirable modes. (c) Strategies that can increase the probabilities of desired modes and facilitate transitions to overcome locomotor challenges. (i–v) Model systems.
For both robots and animals, feed-forward self-propulsion, and resulting passive mechanical interaction, destabilizes the system and induces stochastic, barrier-crossing locomotor transitions. In addition, animals also use feedback-controlled active adjustments to facilitate or enable desired transitions when feed-forward self-propulsion is insufficient.
Based on these insights, we discovered a suite of strategies to make desired transitions more probable for each model system (Figure 2c). This owes to the fact barrier-crossing transitions on landscape can be enhanced or suppressed by steering the system state on the energy landscape, changing landscape barriers, or even modifying landscape topology (the number of basins). These strategies increased robot performance or even enabled new capabilities.
Finally, these results showed that there is a potential energy landscape-dominated regime, in which self-propulsion does not guarantee overcoming potential energy barriers. In this regime, our approach is powerful for understanding how to self-propel to make locomotor transitions.
Towards multi-pathway locomotor transitions
Considering the generality of physical principles across diverse simple model systems, we posit that multi-pathway locomotor transitions in heterogeneous terrain can be understood by composing larger scale, higher dimensional potential energy landscapes from simple landscapes. Furthermore, potential energy landscape theories with the addition of intelligence can help understand and predict how the animal’s nervous system or robots’ sensing, control and planning systems mediate physical interaction to generate multi-pathway locomotor transitions. Such predictive energy landscape approaches will complement existing geometry-based obstacle avoidance approach in robotics (Figure 1a-c) and help robots better use physical interaction to traverse currently unreachable complex 3-D terrain.
Related publications
- Othayoth R, Xuan Q, Wang Y, *Li C (2021), Locomotor transitions in the potential energy landscape-dominated regime, Proceedings of the Royal Society B: Biological Sciences, 288 (1949): 20202734 PDF (Movie, Video abstract, Talk)
- *Li C, Pullin AO, Haldane DW, Lam HK, Fearing RS, Full RJ (2015). Terradynamically streamlined shapes in animals and robots enhances traversability through densely cluttered terrain, Bioinspiration & Biomimetics, 10 (4), 046003 (B&B Highlights of 2015) PDF
- Othayoth R, Thoms G, *Li C (2020), An energy landscape approach to locomotor transitions in complex 3-D terrain, Proceedings of the National Academy of Sciences, pnas.1918297117, PDF (Movies 1 2 3 4 5 6 7 8 9 10).
- Gart SW, *Li C (2018). Body-terrain interaction affects large bump traversal of insects and legged robots, Bioinspiration & Biomimetics, 13, 026005 PDF (Movies 1 2 3 4)
- Gart SW, Yan C, Othayoth R, Ren Z, *Li C (2018). Dynamic traversal of large gaps by insects and legged robots reveals a template, Bioinspiration & Biomimetics, 13, 026006 PDF (Movies 1 2 3 4)
- Han Y, Othayoth R, Wang Y, Hsu C-C, de la Tijera Obert R, Francois E, *Li C (2021), Shape-modulated obstacle attraction and repulsion during dynamic locomotion, International Journal of Robotics Research, doi: 10.1177/0278364921989372 PDF (Movies 1 2 3 4 5 6 7 8 9 10 11 12 13)
- *Li C, Wöhrl T, Lam HK, Full RJ (2019). Cockroaches use diverse strategies to self-right on the ground, Journal of Experimental Biology, 222 (15), jeb186080 PDF (Movies 1 2 3)
- Othayoth R, *Li C, Co-opting propelling and perturbing appendages facilitates strenuous ground self-righting, eLife, in revision
- *Li C, Kessens CC, Fearing RS, Full RJ (2017). Mechanical principles of dynamic terrestrial self-righting using wings, Advanced Robotics, 31, 881-900 (Invited Paper; Advanced Robotics Best Paper Award, 4 awarded out of 96) PDF
- Xuan Q, *Li C (2020). Coordinated appendages help accumulate energy to self-right on the ground, IEEE Robotics and Automation Letters, 5 (4), 6137-6144 (also appearing in IROS 2020) PDF Video IROS pre
- Xuan Q, *Li C (2020). Randomness in appendage coordination facilitates strenuous ground self-righting, Bioinspiration & Biomimetics, 15 (6), 65004 PDF (Movies 1 2 3)
- Wang Y, Othayoth R, and *Li C (2021), Uncovering the role of head flexion during beam obstacle traversal of cockroaches, Integrative and Comparative Biology, 61,p. e959